Click here to read Part 1 of this blog.
By Wlodzimierz S. Erdmann, Piotr Aschenbrenner, Vasilis Giovanis
To prevent sport accidents, one needs to understand injury mechanisms [8]. At the end of the 20th century and beginning of the 21st century huge investigations were taken out on alpine skiing using several methods to understand the tactics of running and the influence of fatigue on accidents [9-11]. The new and improved methods and instruments used in alpine ski research are listed here:
- photo-optical methods
- image-based methods
- radio methods
- electro-mechanical sensors
- other new methods and instruments.
The first part of this blog was devoted to photo-optical, video-based and part of radio methods (radar). We continue explaining the methods below:
3) Radio methods – continued
Global navigation satellite system provides users with positioning, navigation, and timing (PNT) services. The system consists of three components: 1) the space component (satellites or space vehicles, SV), 2) the control component (tracking stations around the world), and 3) the user component (receivers). Twenty-four satellites are needed to cover the whole Earth; at present there are 30 operational satellites in the global positioning satellite (GPS) constellation plus about 10 in reserve [12].
GPS satellites broadcast radio signals giving information about a) their location, b) their status, c) the precise time (from on-board atomic clocks). GPS radio signals travel through space at the speed of light (c), i.e. at 299,792.458km/s. A GPS device receives the radio signals, notes their exact arrival times and uses this data to calculate its distance from each satellite in view. Once a GPS device knows its distance from at least four satellites, it can use geometry to determine its location on Earth in three dimensions. The signal’s travel time is the difference between the time broadcast by the satellite (t1) and the time the signal is received (t2). The distance from receiver to satellite can be obtained by multiplying the travel time (t2 – t1) by c. The SVs transmit two microwave carrier signals [12].
Separate instrumentation, namely GPS together with the GLONASS (Globalnaia Navigatsionnaia Sputnikova Sistema) of Russian Federation, Galileo of the European Union, COMPASS (BeiDou) of China, and QZSS (Quasi Zenit Satellite System) of Japan compose Global Navigation Satellite Systems (GNSS). At present only GPS and GLONASS are fully operational. Some receiving devices can detect signals from more than one system, in practice the GPS and GLONASS, see Fig. 3.
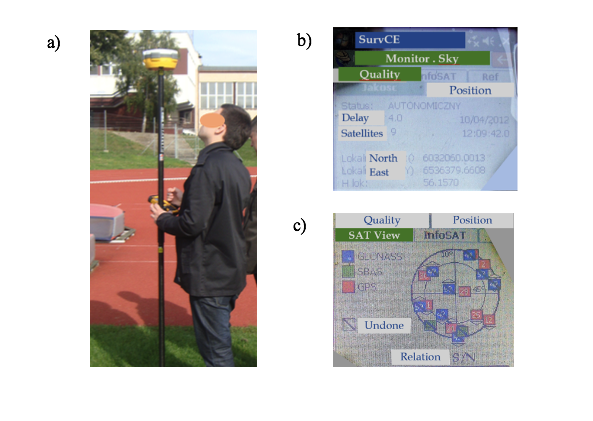
Measurements of the phase of the signal’s carrier wave are used to enhance the precision of position data derived from satellite-based positioning systems. Real Time Kinematic satellite navigation (GNSS-RTK) is a technique based on the carrier wave of a signal. RTK systems use a single base station receiver and a number of mobile units. The nominal accuracy of this system is 1cm horizontally and 2cm vertically [13].
4) Electro-mechanical sensors
Motion trackers are used to obtain real-time information about the movement of objects. Such devices can be used to estimate orientation, position or specific variables such as velocity and acceleration in the navigation frame. Advances in micro-machined electromechanical system (MEMS) technology have enabled the development of low cost, small form factor accelerometers and gyroscope sensor components. To ensure the availability of reliable, accurate tracking estimates MEMS-based inertial measurement unit (IMU) signals need to be combined with measurements from aiding sensors such as magnetometers, barometric-altimeters and global navigation satellite systems [14].
Accelerometers work on the principle that the length of a spring changes according to the forces acting on it. The source of the force is a mass connected to the spring which can accelerate positively (causing the spring to expand) or negatively (causing the spring to compress). A gyroscope is an instrument used for measuring angular motion. Mechanical gyroscopes rely on conservation of angular momentum. When rotated a vibrating element (vibrating resonator) experiences secondary vibration orthogonal to the direction of the original vibration (the Coriolis effect). The rate of turn can be derived from measurements of the secondary vibration. Various micro-mechanical machined geometric devices are available, many use the piezo-electric effect to emit and detect vibration [15].
Magnetic motion capture systems use sensors placed on the body to measure the magnetic field generated by a transmitter source (Fig. 4). The transmitter source consists of three perpendicular coils that emit a magnetic field when a current is applied. The 3D sensors measure the strength of the field, which is proportional to the distance of each coil from the field emitter assembly.
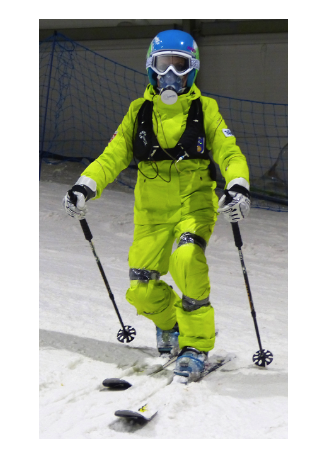
5) Other research methods suitable for alpine skiing
Erdmann [16] has written about the possibility of using robots in sport environments. Nemec and Lahajnar [17] presented a ski robot which was capable of autonomous skiing on a ski slope using the carving skiing technique.
Another new device is the unmanned aerial vehicle (UAV, drone), i.e. a flying object without a pilot on board. This type of aircraft can be controlled remotely by a pilot at a ground control station or fly autonomously based on a pre-programmed flight plan or more complex dynamic automation systems. An UAV equipped with a high-resolution camera makes it possible to capture real-time overhead footage. This can be used to record gate settings or a skier’s run – Fig. 5.
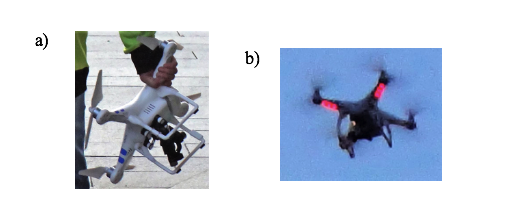
***
Wlodzimierz Stefan Erdmann: Professor of biomechanics at the J. Sniadecki University of Physical Education and Sport in Gdansk, Poland. Areas of interest: human morphology, especially geometry and inertia, myo-mechanics, biomechanics of sport locomotion, specifically tactics of load distribution, also bioengineering and forensic sciences. Scientific consultant to the best judo competitors (Poland, Kuwait), long distance runners (Ethiopia), sprinters (Jamaica), alpine skiers (Poland, Austria) and sport games (Poland).
Piotr Aschenbrenner: Lecturer at the J. Sniadecki University of Physical Education and Sport in Gdansk, Poland. Interested in movement analysis, especially sport technique, muscle strength measurements, computer sciences in physical culture. A scientific consultant to Polish sport associations – sailing, bobsleigh & skeleton, coaches and instructors of skiing. Also a sailing and alpine skiing coach.
Giovanis Vasilios Associate professor of skiing at the School of Physical Education and Sport Science of National and Kapodistrian University of Athens, Greece. Specific interests include the base of race’s skiing biomechanics (alpine skiing and cross country skiing) and, theory and action of coaching and simulation in skiing. Also embrace the topographic and kinematics analysis of race skiing, the comparative analysis of their tactics, and the epidemiology of injuries in these sports.
Competing interests
None
References
- Bahr R, Krosshaug T. Understanding injury mechanisms: a key component of preventing injuries in sport. Br J Sports Med 2005; 39: 324-329.
- Giovanis V. Kinematics of running of alpine skiing courses and problem of injuries. Doctoral dissertation, B. Czech University of Physical Education, Krakow.
- Aschenbrenner P. Kinematics of competitors’ running of alpine skiing giant slalom and its geometrical parameters. Doctoral dissertation, J. Sniadecki University of Physical Education and Sport, Gdansk.
- Erdmann WS, Aschenbrenner P. Biomechanics of tactics of running a course in four alpine skiing disciplines: First course geometry results. Hum Mov, 2008;9:124-7.
- GPS.GOV. Official U.S. Government information about the Global Positioning System (GPS) and related topics. Available: www.gps.gov. Accessed 01 Jul 2018.
- Real Time Kinematics. Available: en.m.wikipedia.org/wiki/Real_Time_Kinematic. Accessed 24 Jun 2018.
- Vydhyanathan A, Bellusci G. The next generation Xsens motion trackers for industrial applications. Xsens 2015. Available: www.xsens.com/ download/pdf/documentation/mti/mti_white_paper.pdf. Accessed 25 Jun 2018.
- Roetenberg D. Inertial and magnetic sensing of human motion. Doctoral dissertation, University of Twente, 2006.
- Erdmann WS. Problems of sport biomechanics and robotics. Int J Adv Robotic Sy, 2013, DOI: 10.5772/52499.
- Nemec B, Lahajnar L. Control and navigation of the skiing robot. The 2009 IEEE/RSJ Int. Conf. on Intelligent Robots and Systems, 11-15 Oct 2009, St. Louis, MO, p. 2321-6.
https://blogs.bmj.com/bjsm/2018/09/23/methods-for-acquiring-kinematic-data-in-alpine-skiing-part-1/