By Wlodzimierz S. Erdmann, Piotr Aschenbrenner, Vasilis Giovanis
The motion involved in alpine skiing is one of the most difficult to analyze because the trajectory is curvilinear and the athlete moves in a wide, open space. In alpine ski disciplines, the skier is moving relative to the start and finish planes, relative to snow/ground level (up and down body motion), and relative to the gates (left and right motion) [1]. Analysis of alpine skiing deals with three main topics: 1) terrain (slope) geomorphology: a) free of snow for homologation, i.e. approval of a slope, b) with snow for competitions; 2) course setting; 3) biomechanics [2]. The examples of course setting modelling are presented in Figure 1.
A valid and practical measurement system for capturing skiers’ kinematics should: 1) be accurate, 2) cause minimal interference with skiing movements, 3) permit capture of the largest possible volumes of training and competition data [4]. It takes into account also the possibility of analyses of slope geomorphology, setting of gates, skier’s kinematics and kinetics.
Previously an article we wrote dealt with historical technological approaches [5]. The aim of this study was to describe the methods and instrumentation currently used in alpine ski research on slope geomorphology, course geometry and course runs. The accuracy of instrumentation and use of instruments by analysts and coaches during training and competition was also investigated. The new and improved methods and instruments used in alpine ski research at the beginning of the 21st century are listed below:
- photo-optical methods
- image-based methods
- radio methods
- electro-mechanical sensors
- other new methods and instruments.
Photo-optical method
In alpine skiing inter-gate distances can be measured using a laser. It is very accurate, with a resolution of up to 0.5-1.0mm over the distances of the order of 100m [6]. In some circumstances coaches can use the Leica Laser Locator. This device has four basic functions: binocular magnification, digital laser rangefinder, digital compass and inclinometer. The user must follow these operational guidelines: use solid reflective objects; do not aim at oblique surfaces or very tall, nearby objects; do not use in moisture atmospheric conditions; do not use in an environment with a high level of vibrations or where there is a risk of occurrence of lightning. The instrument should not be used within 10m of moving objects such as trains or cars, within 2m of large metal objects such as city lamp poles, or within 0.5m of metal tools [6].
A theodolite is a surveying instrument used for measuring angles in the horizontal and vertical planes. Another device, namely the electromagnetic (electronic) distance measurement (EDM) device, can be based on the following sources a) microwaves (unaffected by visibility), b) light waves (visible light – laser), c) infrared wave (use is limited by rain, fog, airborne particles). A device which integrates a theodolite with an EDM device and a computer or microprocessor is called tachymeter or total station. Digital tachymeters allow the user to view information directly via a viewing panel which displays data on: distance, angles, height and spatial co-ordinates of the observed point.
Image-based methods
Image-based methods (photography, video) can be used on their own to obtain information about the location of a skier (according to reference system) and his/her displacement relative to a fixed point. They can also serve as a reference methods when evaluating the accuracy of other methods. Multiple image exposures (e.g. serial photography or video recordings) can be used to obtain data on movement time and derivatives. With sophisticated cameras good photographs can be taken in low light conditions, focusing can be automated and multiple images can be captured with one button push. Images can be used to assess an alpine skier’s movement technique i.e. localization of the skier’s body parts and equipment and centre of mass and the distance of the skier’s centre of mass from the turning pole, ground (snow) or the tip of the ski.
Video images obtained from a camera mounted on the helmet of a fore-runner or images of a competitor skiing the course can be combined with time data for assessment of tactics i.e. for analysis of the line to take between gates and velocity distribution along the course [7], see Fig. 2.
Radio methods
Radar (RadioDetection And Ranging) uses radio waves which are emitted from a transmitter and reflected from a distant object back to the receiver. The distance from the transmitter to the object can be calculated from the velocity of the electromagnetic wave and the time taken for the wave to travel to and from the object.
There are also radar devices that exploit the frequency shift (Doppler shift) in radio waves caused by moving objects, these are called Doppler radar. If an object is moving towards or away from the receiver the frequency of the reflected radio waves will be different from that of the emitted waves. This allows the velocity of an object e.g. a skier to be calculated moving in an environment containing other objects moving at different velocities (using the continuous-wave radar). Objects moving parallel to the radar beam produce the maximum Doppler frequency shift.
In alpine skiing, radar can be used over the parts of downhill courses where skiers achieve very high velocities in order to reduce the risk of accidents. On some courses competitors in alpine skiing World Cup events can achieve speeds of over 42m/s (150km/h) when the snow is hard and visibility is good. During speed skiing competition racers achieve speeds of up to 70m/s (250km/h).
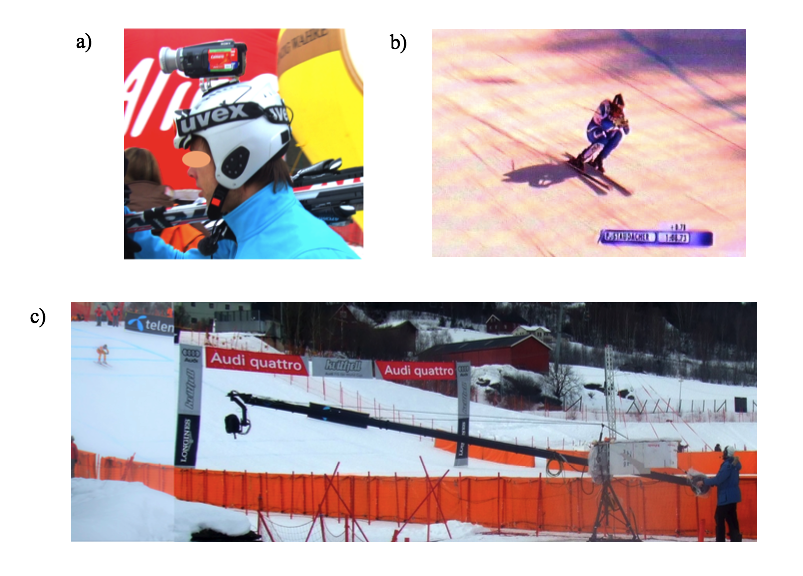
***
Wlodzimierz Stefan Erdmann: Professor of biomechanics at the J. Sniadecki University of Physical Education and Sport in Gdansk, Poland. Areas of interest: human morphology, especially geometry and inertia, myo-mechanics, biomechanics of sport locomotion, specifically tactics of load distribution, also bioengineering and forensic sciences. Scientific consultant to the best judo competitors (Poland, Kuwait), long distance runners (Ethiopia), sprinters (Jamaica), alpine skiers (Poland, Austria) and sport games (Poland).
Piotr Aschenbrenner: Lecturer at the J. Sniadecki University of Physical Education and Sport in Gdansk, Poland. Interested in movement analysis, especially sport technique, muscle strength measurements, computer sciences in physical culture. A scientific consultant to Polish sport associations – sailing, bobsleigh & skeleton, coaches and instructors of skiing. Also a sailing and alpine skiing coach.
Giovanis Vasilios Associate professor of skiing at the School of Physical Education and Sport Science of National and Kapodistrian University of Athens, Greece. Specific interests include the base of race’s skiing biomechanics (alpine skiing and cross country skiing) and, theory and action of coaching and simulation in skiing. Also embrace the topographic and kinematics analysis of race skiing, the comparative analysis of their tactics, and the epidemiology of injuries in these sports.
Competing interests
None
References
- Erdmann WS. Geometry of setting of alpine skiing slalom. Technical Report 1995.02. Dept. of Biomechanics, J. Sniadecki Higher School of Physical Education, Gdansk.
- Gilgien M. Characterization of skier’s mechanics, course setting and terrain geomorphology in World Cup alpine skiing using Global Navigation Satellite System. Injury risk, performance and methodological aspects. Doctoral dissertation, Norwegian School of Sport Sciences, 2014.
- Spörri J., Kröll J., Schwameder H., Schiefermüller Ch., Müller E. Course setting and selected biomechanical variables related to injury risk in alpine ski racing: an explorative study. Br J Sports Med 2012; 46: 1072-7.
- Gilgien M, Spörri J, Limpach P, Geiger A, Müller E. The effect of different global navigation satellite system methods on positioning accuracy in elite alpine skiing. Sensors (Basel), 2014; 14: 18433-53.
- Erdmann WS, Giovanis V, Aschenbrenner P, Suchanowski A, Kiriakis V. Methods for acquiring data on terrain geomorphology, course geometry and kinematics of competitors’ runs in alpine skiing: a historical review. Acta Bioeng Biomech, 2017; 19: 69-79.
- Laser Locator. Operating Instructions. Leica Geosystems AG. Defence & Special Projects, Heerbrugg, CH, 2001. Available: w3.leica-geosystems. com/downloads123/zz/gpsgis/laser-locator/manuale/LL_manual_en.pdf. Accessed 26 Jun 2018.
- Erdmann WS, Giovanis V. Investigations on kinematics of giant slalom’s tactics in alpine skiing. In: Miyashita M, Fukunaga T (eds.) XVI International Congress of Biomechanics, The University of Tokyo, Tokyo, 1997; 79.